Air Traffic Control
ensures that aviation is safe.
Intelligent Decision Support Tools (DSTs)
Air Traffic Control is a human-centered operation ensuring safe, efficient and sustainable flows of traffic
Decision Support Tools are expected to evolve from being used solely as software tools to becoming autonomous agents that work together with Air Traffic Controllers. They can support the Controllers by extending their effective time-horizon, if possible, covering full gate-to-gate operations.
To improve human-machine cooperation, automation should be sensitive and adaptable to individual preferences in problem-solving. Decision Support Tools must align with this. Situations like misunderstanding of what? “it” is doing or why? must be avoided.
DST wishlist:
=> Generate advice compatible with human control strategies. Complex Artificial Intelligent approaches may conflict with this.
=> Reduce controller workload, so minimize:
- false alerts;
- missed alerts;
- monitoring or input requirements.
can make air traffic operations more
efficient and sustainable,
especially in high-density traffic conditions.
Quantify the potential advantages of Trajectory Based Operations (TBO)
Spoiler
For traffic converging into an extended Terminal Area of a main port, Trajectory Based Operations (TBO) could bring 20-25% reduction in fuel consumed [Potential fuel consumption savings in medium to high-density extended terminal areas, A. Benoit et Al., 1983]
The study
In the early 1980s, EUROCONTROL and the ANSPs of the United Kingdom and Belgium joined efforts to estimate the potential advantages from the introduction of TBO. During peak traffic periods, the radar tracks from traffic inbound to London-Heathrow and Brussel National were collected. The data covered the final part of the cruise phase and the full descent.
The traffic sample
The traffic sample of traffic into London Heathrow comprised a 2-hour period during which 46 aircraft landed on Runway 25R.
At the time, the management of the flows in the Terminal Area was facilitated through path stretching and holding circuits as required.
Transit scenarios
The study estimated the fuel burnt by the aircraft in the samples assuming different transit scenarios:
- A hypothetical scenario where each aircraft would be accommodated on its preferred flight profile.
- The actual trajectories flown as recorded by the radars.
- A hypothetical TBO scenario where each aircraft would be allocated the same RTA constraint on the runway as recorded for the actual flight but would make maximum use of the cruise-descent speed range in its operational flight envelope.
Estimates of fuel burnt
A method has been developed for the estimate of the fuel consumption of an aircraft based on the observed trajectory, meteorological data, and an estimate of the landing mass [Method for estimating actual fuel consumption from ground observations, P.Sauer et Al, 1983]
The method covers the enroute climb, cruise and descent, acceleration and deceleration phases, and takes into account particular effects such as airframe configuration changes.
Estimated fuel consumption for different transit scenarios
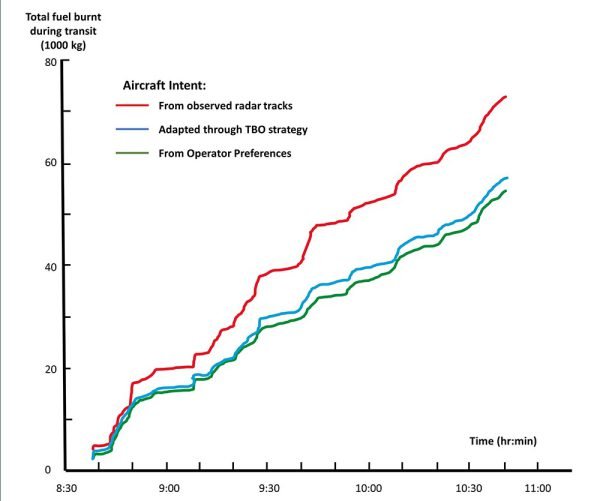
Estimated from radar observations
The red curve depicts the estimated amount of fuel burnt by the aircraft inbound to London-Heathrow as observed by the London radar.
The total amount of fuel consumed was estimated at some 73 tons.
Applying a TBO strategy
The blue curve represents the estimate of the fuel burnt assuming the application of speed control during the final cruise part and the enroute descent.
The aircraft were assumed to follow the standard inbound route. For each flight, the RTA was identical to the one from the radar observations. Transit time that could not be absorbed through speed control was spent through low-altitude vectoring.
The pilot’s preferred profile
Generally, a pilot would aim to fly in accordance with the airline’s recommended cruise-descent speed profile. This would ensure execution of the flight at minimum cost.
In the study, the preferential profile was considered to be the observed profile until this was clearly affected by ATC intervention.
The total amount of fuel burnt was estimated at some 55 tons.
The aircraft was assumed to follow the standard inbound routing without ATC intervention. This holds that the traffic sample was not free of conflict situations and that the sequence of landing times was different from the other transit scenarios.
Fuel savings
The introduction of Trajectory Based Operations using cruise-descent speed control during the final parts of the flight has the potential of saving some 20-24% of fuel consumed using the enroute speeds as control parameters. When using “altitude” as an additional control parameter even greater savings could be achieved.
Safety
The application of enroute speed control creates a natural separation among flights in the arrival streams. When comparing the number of potential loss-of-separation situations in the “TBO” and “Pilot-Preferred” scenarios, a reduction of more than 60% in potential conflicts was observed in the TBO scenario.
Using “altitude” as an additional control parameter would reduce the controller workload even further.
Conclusion
The implementation of Trajectory Based Operations has great potential for improving the safety, efficiency, and sustainability of ATC.
Every Kilo of fuel saved, is not turned into greenhouse gasses and does not generate noise in the burning process.
Meet the actors:
The Aircrew:
Plan your flight – Fly your plan
The “plan”, aka Aircraft Intent, describes the planned route, altitude profile and sequence of airspeeds used.
The “preferred” Aircraft Intent assumes that the flight is not affected by external disturbances.
The Air Traffic Controllers:
The Decision-Making process of Controllers is based essentially on the prediction of the medium-term future.
This is facilitated by information ……..
- provided before the flight,
- sensed by ground-based installations,
- transponded from aircraft,
- computed from collated data,
- communicated by aircrew and other Controllers.
If the predicted future seems unsatisfactory, then remedial action is undertaken by requesting the Aircrew to update the Aircraft Intent through Clearances.
The Decision Support Tools:
Decision Support Tools (DST) provide the Controllers with better support for making their decisions.
They require access to similar information as the Air Traffic Controllers.
Setting the scene
Setting the scene ….
The Air Traffic Control system is a complex, human-centered system comprising a wide variety of people and machines with many different competencies and capabilities.
The safe operation of aircraft is managed by the Aircrews.
The Ground System is best equipped for managing the traffic flows optimizing Safety, Efficiency and Sustainability.
The European Organization for the Safety of Air Navigation, EUROCONTROL, has been at the forefront of R&D activities to improve Safety, Efficiency and Sustainability in Air Traffic Management.
R&D into Decision Support Tools at EUROCONTROL HQ from the end of the 1960s.
The tools evolved from straightforward “Conflict Alert” tools to the TMA 2010+ toolset based on the “Best Next Clearance” concept using Augmented Intelligence strategies.
Conflict Alert
(CA)
The Conflict Alert (CA) function alerts Controllers for potential conflict situations within the target time horizon, e.g., covering the extent of their control sector.
A potential conflict is detected if the predicted separation between two flights is below a safe minimum.
Strategy
For each aircraft in the control zone a trajectory is predicted based on the published Aircraft Intent, predicted meteorological conditions and aircraft performance.
Caveat
Uncertainties in the actual Aircraft Intent applied, meteorological conditions and aircraft performance result in uncertainties in the predicted aircraft positions. This results in numbers of Missed and False Alerts presented to the Controllers that increase with the look-ahead time and traffic density
The increased controller workload makes Medium term conflict detection only effective in low to medium complexity enroute sectors.
Note: Decision support tools in operation today barely extend beyond safety net features providing last-minute alerts for evasive actions.
The way forward
Develop a Trajectory Based Strategy that reduces the number of potential conflicts by adapting the Aircraft Intent at entry into the control zone. This reduces the controller workload by reducing the False Alert rate.
Conflict Detection & Resolution
(CDR)
When the Conflict Alert module is complemented with a Conflict Resolution module, it will be possible to create conflict-free paths for the aircraft through the control zone. This reduces the controller workload significantly.
Strategy
At entry in the control zone, the Conflict Resolution module will compute for each aircraft an updated Aircraft Intent by adding additional altitude and radar vector clearances so that the potential conflict situations will be resolved.
Caveat
The uncertainty in the predicted aircraft positions increases with the look-ahead time. This forces the Conflict resolution module to apply separation criteria that are much greater than the minimum required radar separations.
The increased controller workload makes Medium term conflict detection only effective in low to medium complexity enroute sectors.
Increasing traffic complexity significantly reduces sector capacity and flight efficiency.
Consequently, the project was shelved after the International Conference on Automatic Conflict Detection and Resolution in Luxembourg, 1976
The way forward
Potential conflicts occur at points where traffic converges. Develop a Trajectory Based Strategy that ensures that predicted trajectories will achieve minimum radar separation at the convergence point. This reduces potential conflict situations and optimizes control sector capacity.
Time-Based Flow Management
(TBFM)
The Controllers strategy: Trajectory Prediction – Conflict Detection – Conflict Resolution is perfect for ensuring safety, but less efficient for smoothing traffic streams optimizing efficiency and sustainability. The conclusions from the 1976 Luxembourg seminar hinted on a requirement for a strategy change.
Paradigm change
The Conflict Detection & Resolution strategy starts from a Controller’s perspective:
Predict the future evolution from the “now” situation. Then take remedial action if required.
The limited accuracy of predicted trajectories limits the achievable time horizon in the control sector. In contrast, the TBO approach requires trajectory management over an extended part of the flight, aka multiple control sectors. This requires a paradigm shift.
Strategy
The focus of the traffic management strategy is changed from Conflict Detection to Conflict Resolution. First identify the convergence points of the traffic streams, then build a safe flow through scheduling and sequencing the individual aircraft whilst applying the minimum safety separations.
This defines for each aircraft the 4D-target conditions and thus the Required-Times-of-Arrival (RTAs) at the convergence points.
If the target conditions are different from the ones of the predicted trajectories based on the current Aircraft Intent, then the latter needs to be adapted. Depending on the control strategy and/or aircraft equipment the required control actions can either be performed through clearances by the Controllers or delegated to airborne automation.
Approaches implemented include Arrival Management tools (AMAN) that inform the Controllers about “Time-to-Loose or -Gain” or through negotiating the Required-Time-of-Arrival with the Flight Management System (FMS) in the aircraft.
Caveat
The TBFM strategy facilitates TBO through a negotiation process with all client actors. During the flight, multiple perturbations, e.g., meteorological conditions, availability of resources, Controller clearances, limitations in the operational flight envelop of aircraft, etc., may impact the feasibility of the RTA plan resulting in a stability and workload challenge.
The way forward
These caveats became apparent during a man-in-the-loop experiment in 1982. The validity of basis of the concept, i.e., the practicality of sequencing and sequencing at convergence points could be validated. The control strategy needs to be adapted to mitigate the impact of required variations of the RTAs when adapting the flows to the evolving real-life conditions.
Best Next Clearances
(BNC)
===> to be edited <===
The methodology proposed in this paper consists of an iterative approach that couples optimization and simulation to find solutions that are resilient to perturbations due to the uncertainty present in different phases of the arrival and departure process.
- The human factor: ATC relies heavily on human controllers to make decisions and manage traffic. Human errors can occur, and it is important to have systems in place to detect and mitigate these errors. Furthermore, ATC controllers are facing high workloads and stress, which can lead to fatigue, making them more prone to errors.
Lessons learned
Short term conflict alert is online at UAC Maastricht
The trajectory prediction function can be used in Flight Data processing Systems
Allocating conflict-free trajectories within a medium-term time horizon would result in an unacceptable reduction of sector capacity
EUROCONTROL-CAA project
1982: TBO concept implemented through trajectory synchronisation is not practical due to the impact of real-life perturbations
1980: Trajectory Based Operations have great potential for improving safety, efficiency and sustainability.
EEC simulation
1989: TBO concept implemented through air-ground synchronization of Aircraft Intent proved to be efficient .
Mode S experiment
2001: ARETA project proved that TBO through synchronization of aircraft Intent is compatible with existing Flight Data Processing Systems
integration at Belgocontrol
2003:FAA/EUROCONTROL COOPERATIVE R&D – Common Trajectory Prediction Capability
need
collaboration
DASC
2009: EUROCONTROL demonstrated the compatibility of air-ground synchronization of trajectories in low-medium density traffic and Aircraft Intent in high-density traffic conditions.
Global 09 demonstration
Lessons ignored
PHARE
Garteur
Concept
PD3
emperor sesar
Modal Box Title
Modal Box Content
EATCHIP/EATMP
SESAR-JU
datalink: focussed on air-ground trajectory synchronization
ASA focussed on TBO through trajectory synchronization (AMAN)
mention COMPAS, Maestro, CTAS
Validation programs became expensive so EU funding was required
EATMP progress unsatisfactory
Unsolicited proposal
Objectives
Based on PHARE results
Results in closing down EUROCONTROL R&D activities
Where are we now?
Heathrow story
1980 traffic
first managed through flight path extension. entry rate was appr 24 ac/hr. Around 9:20 the entry rate increased to 36 ac/hr. The controllers switched to managed the flow through the holdings.